The first antibiotic that didn’t work for Debbi Forsythe was trimethoprim. In March 2016, Forsythe, a genial primary care counsellor from Morpeth, Northumberland, contracted a urinary tract infection. UTIs are common: more than 150 million people worldwide contract one every year. So when Forsythe saw her GP, they prescribed the usual treatment: a three-day course of antibiotics. When, a few weeks later, she fainted and started passing blood, she saw her GP again, who again prescribed trimethoprim.
Three days after that, Forsythe’s husband Pete came home to find his wife lying on the sofa, shaking, unable to call for help. He rushed her to A&E. She was put on a second antibiotic, gentamicin, and treated for sepsis, a complication of the infection that can be fatal if not treated quickly. The gentamicin didn’t work either. Doctors sent Forsythe’s blood for testing, but such tests can take days: bacteria must be grown in cultures, then tested against multiple antibiotics to find a suitable treatment. Five days after she was admitted to hospital, Forsythe was diagnosed with an infection of multi-drug-resistant E coli, and given ertapenem, one of the so-called “last resort” antibiotics.
It worked. But damage from Forsythe’s episode has lingered and she lives in constant fear of an infection reoccurring. Six months after her collapse, she developed another UTI, resulting, again, in a hospital stay. “I’ve had to accept that I will no longer get back to where I was,” she says. “My daughter and son said they felt like they lost their mum, because I wasn’t who I used to be.” But Forsythe was fortunate. Sepsis currently kills more people in the UK than lung cancer, and the number is growing, as more of us develop infections immune to antibiotics.
Antimicrobial resistance (AMR) – the process of bacteria (and yeasts and viruses) evolving defence mechanisms against the drugs we use to treat them – is progressing so quickly that the UN has called it a “global health emergency”. At least 2 million Americans contract drug-resistant infections every year. So-called “superbugs” spread rapidly, in part because some bacteria are able to borrow resistance genes from neighbouring species via a process called horizontal gene transfer. In 2013, researchers in China discovered E coli containing mcr-1, a gene resistant to colistin, a last-line antibiotic that, until recently, was considered too toxic for human use. Colistin-resistant infections have now been detected in at least 30 countries.
“In India and Pakistan, Bangladesh, China, and countries in South America, the resistance problem is already endemic,” says Colin Garner, CEO of Antibiotic Research UK. In May 2016, the UK government’s Review on Antimicrobial Resistance forecast that by 2050 antibiotic-resistant infections could kill 10 million people per year – more than all cancers combined.
“We have a good chance of getting to a point where for a lot of people there are no [effective] antibiotics,” Daniel Berman, leader of the Global Health team at Nesta, told me. The threat is difficult to imagine. A world without antibiotics means returning to a time without organ transplants, without hip replacements, without many now-routine surgeries. It would mean millions more women dying in childbirth; make many cancer treatments, including chemotherapy, impossible; and make even the smallest wound potentially life-threatening. As Berman told me: “Those of us who are following this closely are actually quite scared.”
Bacteria are everywhere: in our bodies, in the air, in the soil, coating every surface in their sextillions. Many bacteria produce antibiotic compounds – exactly how many, we don’t know – probably as weapons in a microscopic battle for resources between different strains of bacteria that has been going on for billions of years. Because bacteria reproduce so quickly, they are able to evolve with astonishing speed. Introduce bacteria to a sufficiently weak concentration of an antibiotic and resistance can emerge within days. Penicillin resistance was first documented in 1940, a year before its first use in humans. (A common misconception is that people can become antibiotic-resistant. They don’t – the bacteria do.)
“Antibiotics have only been around for the last 70 or 80 years. Bugs have been on this planet for 3bn years. And so they have developed all sorts of survival mechanisms,” says Garner.
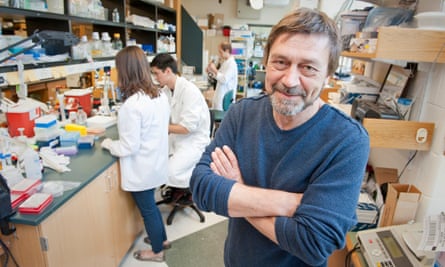
The problem is that today, antibiotics are everywhere, too. One in three of us is prescribed a course of antibiotics each year – a fifth of those needlessly, according to Public Health England. For decades, many farmers have routinely injected livestock with antibiotics, as much to help fatten them up as to prevent infection (this practice is now banned in the EU, US and Canada.) “Our generation is besotted by the powers of antibiotics,” says Jim O’Neill, the economist behind the government’s review. “The problem is we use them for things that we shouldn’t need to.”
In the early decades of antibiotics, resistance wasn’t a serious problem – we’d just find a new drug. After penicillin revolutionised healthcare on the battlefields of the Second World War, the pharmaceutical industry embarked on a golden era of antibiotic discovery. Companies enlisted explorers, missionaries and travellers from around the world to bring back soil samples in the hunt for novel compounds. Streptomycin was discovered in a field in New Jersey; vancomycin, the jungles of Borneo; cephalosporins from a sewage outlet in Sardinia.
But the golden age was short-lived. New discoveries slowed. Antibiotic compounds are common in nature, but ones that can kill bacteria without harming humans aren’t. Soon, big pharma companies began cutting funding to their antibiotic research departments before shutting them down altogether.
“The reality is that we do not have sufficient investment by the private sector to support new research and development,” says Tim Jinks, head of the Drug Resistant Infections programme at the Wellcome Trust. The problem is simple economics: ideally, antibiotics would be cheap, but also used as little as possible. That’s not a great business proposition. And given that antibiotic resistance can emerge as soon as a year after introduction of a new class, a new antibiotic might only have an effective lifespan of 10-15 years – barely enough to pay off years in development. “The numbers just don’t add up,” he says.
There is still hope. In early 2015, researchers at Northeastern University in Massachusetts announced they had discovered a new class of antibiotics in a Maine field. Called teixobactin, it is produced by a newly discovered bacterium, Eleftheria terrae, and effective against a range of drug-resistant infections. Teixobactin was discovered by Slava Epstein and Kim Lewis, using an iChip, an ingenious device about the size of a USB chip designed to overcome a problem that has vexed biologists for decades: of the untold billions of bacteria in nature, only 1% of the species will grow in a Petri dish. “We came up with a simple gadget,” Lewis says. “You take bacteria from soil, sandwich it between two semi-permeable membranes, and essentially trick the bacteria.” So far the pair have identified around 80,000 previously uncultured strains using the device, and isolated several encouraging new antibiotics.
Teixobactin is particularly promising for a simple reason: to date, no bacteria have been able to develop resistance to it. “When we published the paper four years ago, a number of my colleagues wrote me emails saying: ‘Send me teixobactin, and I’ll send you back resistant mutants,’” Lewis says. “I’m still waiting.”
Ishwar Singh remembers the moment he heard about teixobactin: “It was 7 January 2015, on the BBC,” he says. A reader at University of Lincoln’s School of Pharmacy, Singh specialises in developing novel drugs. The news fascinated him. “Most antibiotics target proteins. Teixobactin acts on a lipid – the building block of the cell wall,” he explains. It attacks in several ways simultaneously, making resistance – so far, at least – impossible. Singh shakes his head in awe. “Nature has built such a beautiful molecule.”
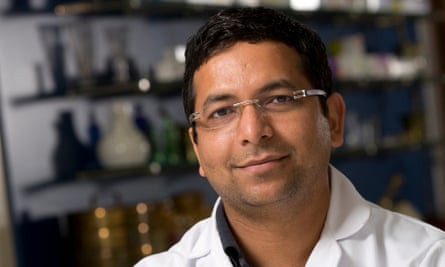
Today, Singh leads one of several teams around the world developing teixobactin. I meet him on a wet morning in January at his lab, where he’s wearing rimless glasses and an expression of keen optimism. On one lab bench, Singh has sketched out teixobactin’s chemical structure in multicoloured board markers. Postdoctoral researchers shuffle around, testing samples for purity. A PhD student holds up a tiny vial containing a thumbnail’s width of fine white powder. “That’s teixobactin,” Singh says.
At first, producing even such a tiny amount proved challenging. Then, in March last year, Singh’s team made a significant breakthrough: they replaced a difficult-to-produce amino acid with another, cheaply available alternative. “There wasn’t much to lose, because people were already saying it wasn’t going to work,” he says. But it did – tests showed it was effective in infections in mice. Singh estimates the new structure will reduce the cost of production 200,000-fold.
Nevertheless, teixobactin is still years away from being tested in humans. Bringing it to market could take a decade or more, if it works at all. Other new drugs are further along: zoliflodacin, intended to treat multi-drug-resistant Neisseria gonorrhoea, is currently in phase three human trials. In 2016, spurred by the growing crisis, the US, UK and charitable organisations including the Wellcome Trust launched the CARB-X initiative, offering $500m in funding for promising new antibiotics. Thanks to techniques like rapid gene sequencing and metagenomics – which searches for promising DNA in the environment, then clones it into new bacteria – scientists have recently discovered a whole host of promising new compounds, including one found inside the human nose. “Things are definitely happening, which is good,” says Lewis. “But it’s a small trickle.”
Given the urgency of the problem, others are taking more pragmatic approaches. One of the most promising is perhaps the simplest: give patients more than one drug at a time. “Everything we use for common infection is monotherapy,” explains Anthony Coates, a professor of medical microbiology at St George’s teaching hospital in Tooting, London. By contrast, combination therapy – using more than one complementary drug in concert – is standard in many other fields. “Aids is one, oncology is another,” he says. “Why aren’t we doing this with common bacteria?”
I meet him at his home in London. He has a quiet, considered manner, which makes his concern all the more alarming. “AMR is a disaster,” he says. “We’re seeing this deterioration happening quicker than I’d ever imagined.”
Coates’s specialism is in so-called antibiotic resistance breakers – compounds that, applied in combination, can make drug-resistant bacteria susceptible to antibiotics again. In 2002 he launched a company, Helperby Therapeutics, to develop combination drugs; several are now in clinical trials. “We screen for thousands of combinations,” he says. Until recently, the work was slow and laborious, done by hand, but advances in robotics and AI are now enabling much of it to be automated, which has allowed more complex combinations.
Exactly why combination therapies work isn’t always clear. “We understand some of the twos: you have a bug, you punch holes in it with one antibiotic, then that allows the second antibiotic in,” Coates says. “When you get three acting together, it’s more complicated. Four and five: very complicated.” But how the combinations work isn’t as important as the fact they do.
One advantage of combination therapy is that many of the drugs Helperby is screening have already been through the extensive clinical trials required before they can be administered to patients – “probably millions of people” – so the likelihood of the drugs failing to pass human trials are lower.
New drugs alone won’t solve the resistance problem. “Yes, it’s important to get new drugs, but it only helps manage the issue for another generation,” O’Neill says. What brought the MRSA epidemic under control was not a drug, but improved hospital hygiene: washing hands. O’Neill’s biggest wish isn’t a treatment at all. “If I was told, ‘You can only have one thing,’ it would be state-of-the-art diagnostics to reduce inappropriate usage,” he says.
Diagnosing whether an illness is caused by bacteria or a virus is one of the most common tasks doctors face, but it’s fiendishly difficult. Symptoms overlap. “The kinds of diagnostic tests that are traditionally used by doctors take a long time and are complex,” explains Cassandra Kelly-Cirino, director of emerging threats at the Geneva-based Foundation for Innovative New Diagnostics. “Most doctors will err on the side of caution and give antibiotics, even though the patient might actually have a virus.” Faced with pushy patients desperate to feel better, it’s often easier (and cheaper) to prescribe a course of penicillin, whether necessary or not.
In 2014, in a bid to develop novel, accessible diagnostic tests, the UK government launched the £8m Longitude Prize, which today is monitoring 83 teams in 14 countries. “Some of the projects are really innovative,” says Nesta’s Daniel Berman, who leads the team of judges. An Australian group is using AI to look at patterns in blood tests to predict sepsis. A team from Pune, India, has developed an ingenious credit-card-sized test called USense to test for UTIs. “You put a urine sample in it, and it tells you which of four antibiotics would be susceptible,” says Berman. Results take 60 minutes. If successful, the USense test could help prevent cases like Debbi Forsythe’s, in which a faster diagnosis could have prevented sepsis.
Making a dent in antibiotic resistance will require such international efforts. Some 90% of forecast deaths from AMR will take place in Africa and Asia – the countries where antibiotic overuse, and resistant infections, are highest. When the AMR review was published in 2016, O’Neill was encouraged by the international response. But since then, Brexit and the Trump administration have knocked AMR off the news agenda. And despite enthusiastic rhetoric, pharmaceutical companies continue to tread water.
“I occasionally think that pharmaceutical company CEOs say to themselves, ‘We’ll just wait until it becomes a real crisis,’” says O’Neill.
Comments (…)
Sign in or create your Guardian account to join the discussion